Effect of Electron Withdrawing Group on Phenyl Boronic Acid for the Synthesis of Indole by Nickle Catalyst
DOI:
https://doi.org/10.33826/journaloms/v05i10.1Keywords:
Fischer indole synthesis, Nuclear Magnetic Resonance (NMR) Spectroscopy, Column Chromatography, Biochemical ImportanceAbstract
Indole is a major heterocyclic system that is indestructible due to its significance in biochemical importance, for example as a component of tryptophan. Indole derivatives have been identified in the literature as having a wide variety of biological characteristics and the immense potential to be used for novel treatments. One of the most proven and trustworthy methods for producing substitute indole is Fischer indole synthesis, which was developed in 1883. Since then a huge number of synthetic methods have been developed, and there a very few methods that can be used to add unsaturation at 3-position of indole. Furthermore, previous methods either required harsh reaction conditions or required the use of challenging substrates to synthesize indole by these conventional methods. In this work, we designed an approach to the synthesis of indole by using Nickle-catalyzed arylatic cyclization for alkenyl substitution at 3-position. Furthermore, the effect of electron–withdrawing groups on phenylboronic acid for the synthesis of indole by nickel catalysis was explored. A suitable substrate was synthesized by a three steps short synthesis involving N-methylation of 2-iodoaniline group at 2-position of derivatized aniline. In the presence of Ni (OAc) 2.4H2O as a catalyst, pyphos as a ligand the 2-alkinylanilid was allowed to react with electron-deficient phenylboronic acid in trifluoroethanol at 800C to give the desired 3-alkyneylindole derivatives. Reaction progress was monitored by TLC and products were purified by column chromatography. The synthesized product was characterized through techniques including infrared spectroscopy and nuclear magnetic resonance (NMR) spectroscopy. These synthesized indole derivatives can be tested to explore their potential as a biologically active agent.
References
• Batail, N., V. Dufaud and L. Djakovitch (2011). "Larock hetero-annulation of 2-bromoanilines with internal alkynes via ligand and salt-free Pd/C catalyzed reaction." Tetrahedron letters 52(16): 1916-1918.
• Gribble, G. W. (2000). "Recent developments in indole ring synthesis—methodology and applications." Journal of the Chemical Society, Perkin Transactions 1(7): 1045-1075.
• Kaushik, N. K., et al. (2013). "Biomedical importance of indoles." Molecules 18(6): 6620-6662.
• Wagaw, S., B. H. Yang and S. L. Buchwald (1998). "A palladium-catalyzed strategy for the preparation of indoles: A novel entry into the Fischer indole synthesis." Journal of the American Chemical Society 120(26): 6621-6622.
• Arcadi, A., et al. (2004). "Gold (III)-catalyzed annulation of 2-alkynyl anilines: a mild and efficient synthesis of indoles and 3-halo indoles." Synthesis 2004(04): 610-618.
• Batail, N., et al. (2011). "Larock hetero-annulation of 2-bromoanilines with internal alkynes via ligand and salt-free Pd/C catalyzed reaction." Tetrahedron letters 52(16): 1916-1918.
• Cheng, H.-G., et al. (2019). "Selective Methylation of Amides, N-Heterocycles, Thiols, and Alcohols with Tetramethylammonium Fluoride." Organic Letters 22(1): 331-334.
• Choi, I., et al. (2016). "Active and recyclable catalytic synthesis of indoles by reductive cyclization of 2-(2-nitroaryl) acetonitriles in the presence of Co–Rh Heterobimetallic nanoparticles with atmospheric hydrogen under mild conditions." Organic Letters 18(21): 5508-5511.
• Coleman, C. M. and D. F. O'Shea (2003). "New organolithium addition methodology to diversely functionalized indoles." Journal of the American Chemical Society 125(14): 4054-4055.
• Dobbs, A. (2001). "Total synthesis of indoles from Tricholoma species via Bartoli/heteroaryl radical methodologies." Journal of Organic Chemistry 66(2): 638-641.
• Gribble, G. W. (2000). "Recent developments in indole ring synthesis—methodology and applications." Journal of the Chemical Society, Perkin Transactions 1(7): 1045-1075.
• He, Z., et al. (2010). "Iodine-mediated synthesis of 3 H-indoles via intramolecular cyclization of enamines." The Journal of Organic Chemistry 75(13): 4636-4639.
• Hiroya, K., et al. (2005). "Mild and efficient cyclization reaction of 2-ethynyl aniline derivatives to indoles in an aqueous medium." Tetrahedron 61(46): 10958-10964.
• Jia, Y. and J. Zhu (2006). "Palladium-catalyzed, modular synthesis of highly functionalized indoles and tryptophans by direct annulation of substituted o-haloanilines and aldehydes." The Journal of Organic Chemistry 71(20): 7826-7834.
• Kaushik, N. K., et al. (2013). "Biomedical importance of indoles." Molecules 18(6): 6620-6662.
• Kondo, T., et al. (2002). "Ruthenium-catalyzed intramolecular oxidative amination of amino alkenes enables rapid synthesis of cyclic imines." Journal of the American Chemical Society 124(2): 186-187.
• Li, Y.-L., et al. (2015). "Metal-free synthesis of indole via NIS-mediated cascade C–N bond formation/aromatization." The Journal of Organic Chemistry 80(8): 3841-3851.
• Liu, B., et al. (2013). "Rhodium (III)-catalyzed indole synthesis using N–N bond as an internal oxidant." Journal of the American Chemical Society 135(44): 16625-16631.
• Liu, F. and D. Ma (2007). "Assembly of conjugated enynes and substituted indoles via CuI/amino acid-catalyzed coupling of 1-alkynes with vinyl iodides and 2-bromotrifluoroacetanilides." The Journal of Organic Chemistry 72(13): 4844-4850.
• McAusland, D., et al. (2011). "The benzyne Fischer-indole reaction." Organic Letters 13(14): 3667-3669.
• Nallagonda, R., et al. (2014). "Synthesis of functionalized indoles via palladium-catalyzed aerobic oxidative cycloisomerization of o-allylamines." Organic Letters 16(18): 4786-4789.
• Ning, X.-S., et al. (2018). "Synthesis of Functionalized Indoles via Palladium-Catalyzed Aerobic Cycloisomerization of o-Allylanilines Using Organic Redox Cocatalyst." The Journal of Organic Chemistry 83(21): 13523-13529.
• Sakai, N., et al. (2008). "InBr3-promoted divergent approach to polysubstituted indoles and quinolines from 2-ethynyl anilines: switch from an intramolecular cyclization to an intermolecular dimerization by a type of terminal substituent group." The Journal of Organic Chemistry 73(11): 4160-4165.
• Selander, N., et al. (2012). "Arylation of rhodium (II) azavinyl carbenes with boronic acids." Journal of the American Chemical Society 134(36): 14670-14673.
• Wagaw, S., et al. (1998). "A palladium-catalyzed strategy for the preparation of indoles: A novel entry into the Fischer indole synthesis." Journal of the American Chemical Society 120(26): 6621-6622.
• Wang, Z., et al. (2019). "Dearomatization–Rearomatization Strategy for Reductive Cross-Coupling of Indoles with Ketones in Water." Organic Letters 21(7): 2302-2306.
• Yu, S., et al. (2017). "The development of a palladium-catalyzed tandem addition/cyclization for the construction of indole skeletons." The Journal of Organic Chemistry 82(7): 3631-3638.
• Zheng, Y.-T., et al. (2021). "Electrocatalytic Dehydrogenative Cyclization of 2-Vinylanilides for the Synthesis of Indoles." The Journal of Organic Chemistry 86(22): 16001-16007.
• Zhou, L. and M. P. Doyle (2009). "Lewis acid catalyzed indole synthesis via intramolecular nucleophilic attack of phenyldiazoacetates to iminium ions." The Journal of Organic Chemistry 74(23): 9222-9224.
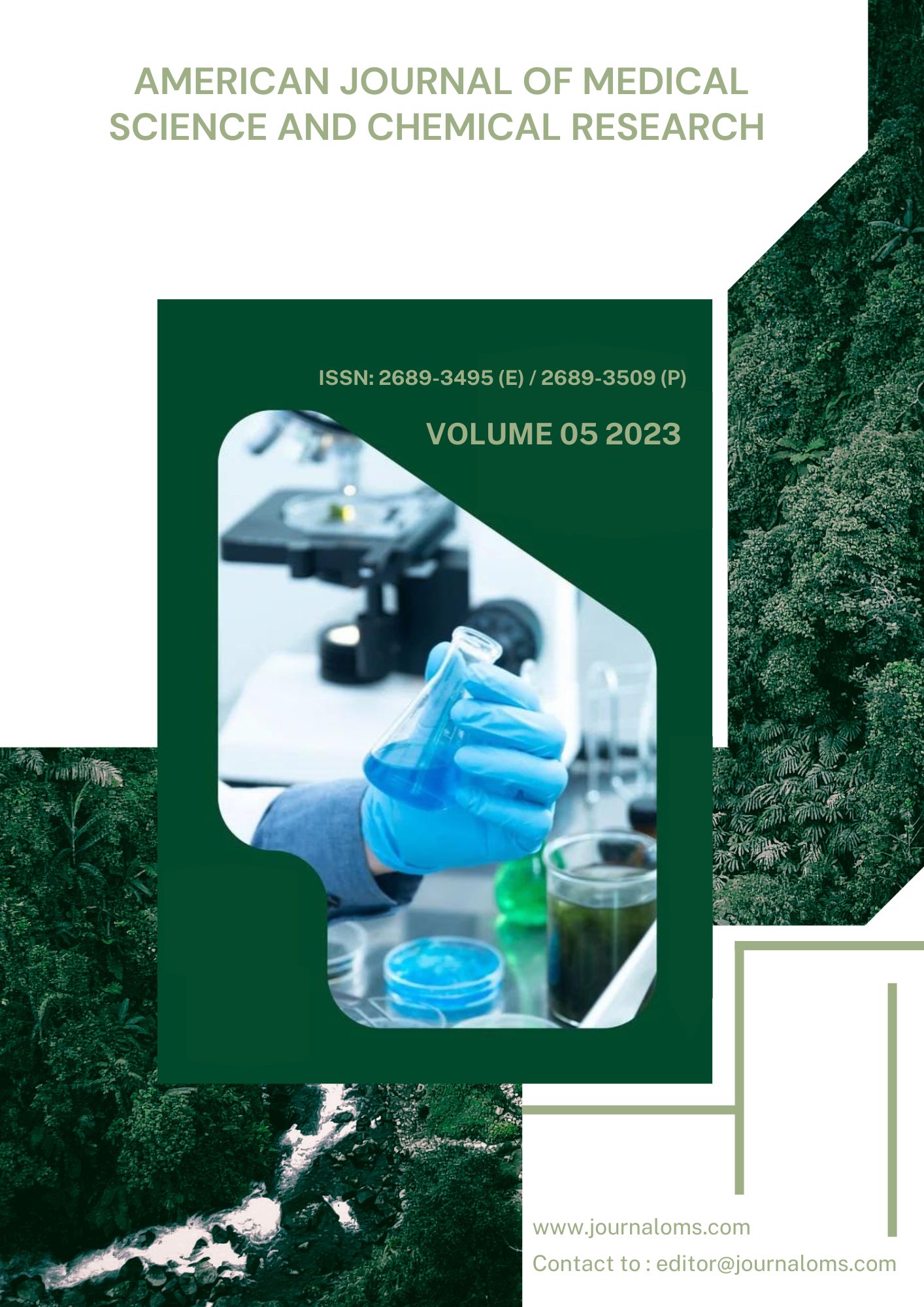
Downloads
Published
How to Cite
Issue
Section
Categories
License
Copyright (c) 2023 Sidra Ghafoor, Ammara Fatima, Mahendra singh Kushwah

This work is licensed under a Creative Commons Attribution 4.0 International License.
All content of this journal: Copyright © 2023 American Journal of Medical Science and Chemical Research (Journaloms), its licensors, and contributors. All rights are reserved, including those for text and data mining, AI training, and similar technologies. For all open-access content, the Creative Commons licensing terms apply.